Techniques for 3D-printed biomedical sensors
Applications | 16 June 2022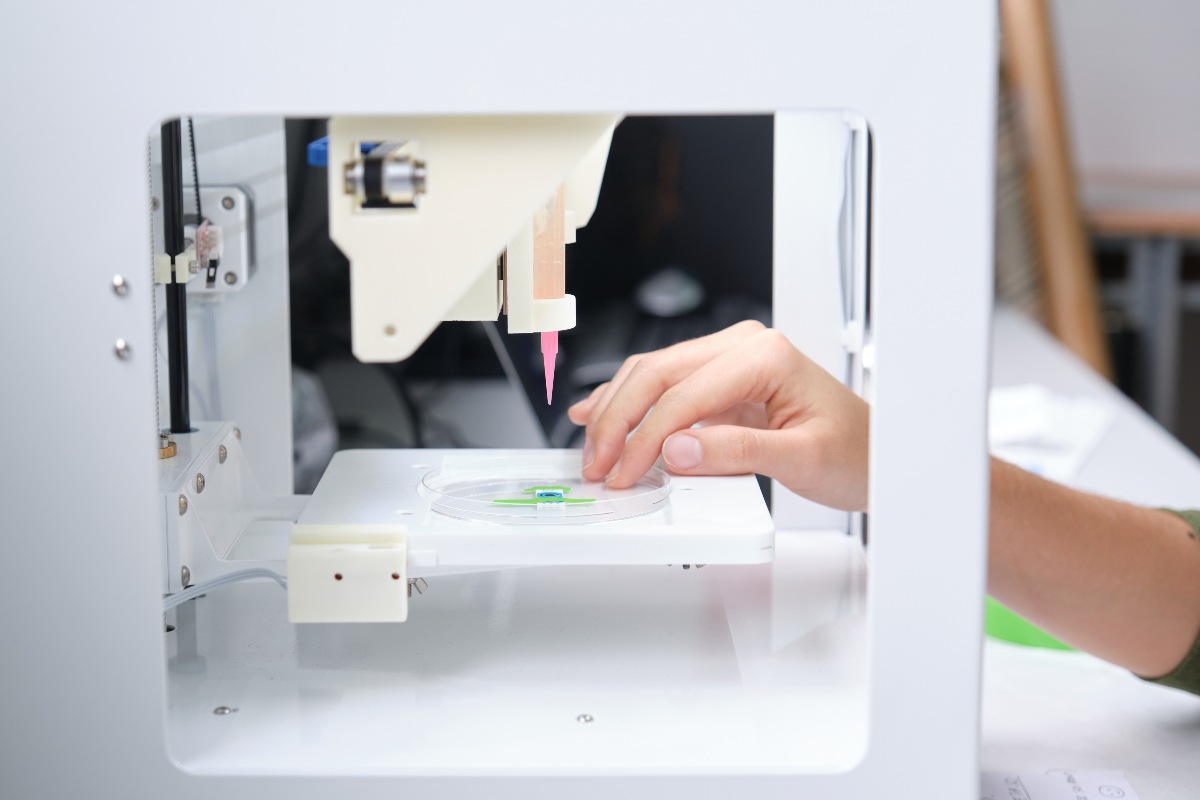
The use of biomedical sensors for the measurement of physiological parameters in humans is now long-established. Micro- and nano-sensors that have been employed so far have been built on silicon-based substrates, but these present many disadvantages in terms of temperature, signal/noise considerations and costs. In addition, most are non-biocompatible, and in the absence of any biocompatibility, they are limited to non-implantable or non-continuous applications.
Flexible sensors that can be fabricated using other materials such as polydimethylsiloxane, polyimide or PET represent a way of overcoming some of those drawbacks and one of the most popular routes to the construction of flexible sensors is 3D printing. The 3D-printed sensors are fabricated by integration of the sensor into a printed platform or by direct printing of the sensing component. One of the attractions of the 3D printing process is that it allows rapid modifications to the sensors where necessary.
Six techniques for 3D printing are covered, and each finds applications in the biomedical sensor area. But each technique has its own advantages and disadvantages. Usually these are attributable to high or low speed, breadth or otherwise of fabrication materials, print accuracy, quality of finish or appearance, suitability or otherwise for large structure creation and cost.
This necessarily brief review focuses solely on stereolithography, its applications, advantages and challenges.
Stereolithography continues to make exciting progress in biomedical sensor production – a reflection of its advantages such as quick fabrication, easy accessibility, the processing of multiple materials and sustainability, as overmatter can be reused. Fewer steps in the sensor fabrication process mean that once a prototype has been uploaded to a system, the production of the sensor takes place without much human intervention. Compared to the other 3D-printing techniques available, stereolithography offers the best possible manufacturing resolution, down to 10μm on two axes and 15μm on the third. This level of resolution makes it ideally suited to the printing of high-quality precise biomedical sensors.
Some of the reported advances for 3D-printed biomedical sensors that have been pioneered through the use of stereolithography alone are as follows:
- A disposable, portable electrochemical sensor for the rapid detection of alkaline phosphatase
- A tethered enzyme capture-sensing system used as a diagnostic tool for malaria
- A carbon quantum dot/genomic DNA complex that can determine levels of DNA damage
- Microfluidic devices capable of sensing the presence of α-fetoprotein antigens
- Microfluidic devices for the rapid detection of E. coli in edible food
- Microfluidic devices for cancer biomarker protein detection
Even with the progress to flexible sensor fabrication and use of plastics as outlined above, the next challenge is the biocompatibility of the materials available for use. The presence of plastic limits many applications in terms of implantability, and prompts questions about reusability and recyclability.
Another limitation that 3D-printed biosensors encounter is emission of harmful and carcinogenic nanoparticles within the human body, which can have disastrous consequences for patient health. This may potentially direct future research towards the use of low-particulate emission materials; some polymers and waxes are already being harnessed in this area, even if the ramifications are a greater number of steps in completing the fabrication process.
Despite its growth, stereolithography in particular is limited by the time required to fabricate each sensor, in addition to the high equipment costs and the fragility of the developed sensors, as fragility is linked to surface roughness. Possible solutions to this challenge may lie in supporting the fragile structures through the incorporation of stronger processing materials such as polydimethylsiloxane.
An allied technique of interest that may be used in conjunction with stereolithography is that of polyjet printing. The two techniques have been used together to produce tissue scaffolds that can provide hierarchical structures where bone simulation is required. However, further work is needed to determine whether the same can be achieved through polyjet printing alone. This process is very valuable for creating multi-material structures. It works through a multi-nozzle process that requires the materials in use to have good shear thinning properties - a limitation; however, the technique offers the advantage of generating large numbers of customisable structures relatively quickly.
A further step envisaged as being instrumental to the development of printed biosensors is the emergence of the 4D printing sector, which represents an enhanced form of these additive manufacturing technologies. It is currently being explored with the use of different materials. The key progression lies in the fact that 4D printing is now employing shape memory polymers so that the fabricated structures respond to external stimuli; the capacity to create more resilient structures of interchangeable morphologies would contribute significantly to further progress in biomedical sensors. Constant changes within the human body and in external environmental factors will drive changes in terms of robustness, sensitivity and morphology, and tantalise scientists with the ideas of converting one-dimensional structures into three-dimensional structures 'origami-style' through responses to external stimuli at any given moment.
In the biomedical sensor sector, an early example of combining 3D printing and 4D printing resides in the area of biomimetics, where applications have employed 3D-printed enzymes. Potentials such as these are making the market for 3D-printed products explode in the current decade. Where the market for 3D-printed materials was placed at $137 million in 2017, as the end of the 20s approaches, it will be touching $4 billion. That is astronomical growth for a technology with spectacular potentials by any standard.